
ANALYSIS OF THE PPARD GENE EXPRESSION
LEVEL CHANGES IN FOOTBALL PLAYERS
IN RESPONSE TO THE TRAINING CYCLE Domańska-Senderowska D, Snochowska A, Szmigielska P, Jastrzębski Z, Jegier A,
Kiszałkiewicz J, Dróbka K, Jastrzębska J, Pastuszak-Lewandoska D, Cięszczyk P,
Maciejewska-Skrendo A, Zmijewski P, Brzeziańska-Lasota E *Corresponding Author: Piotr Zmijewski, Ph.D., Faculty of Medicine, University of Information Technology and Management
in Rzeszow, Rzeszow, Poland. Tel: +48-22-384-08-12. Fax: +48-22-835-09-77. E-mail: zmijewski@op.pl page: 19
|
DISCUSSION
In physical activity, PPARβ/δ acts as a key regulator
of fuel metabolism, promoting a shift from glucose to lipid
as the main energy substrate. It promotes cellular lipid
uptake, activation of fatty acids by fatty acylCoA synthetase
and their mitochondrial uptake and β-oxidation. This
mechanism decreased glucose oxidation as a consequence,
which mimics caloric restriction and physical exercise
conditions. Peroxisome proliferator-activated receptors
play a regulatory role in preventing metabolic disorders
and in muscle adaptation to fasting and physical exercise
[10,21-24]. The studies performed in mice showed
that activation of PPARβ/δ in skeletal muscle results in
enhanced lipid metabolism as an adaptive response to
external stimuli such as food availability and prolonged
physical activity [2,25-27]. This activation of PPARβ/δ in
skeletal muscle enhances lipid use for energy expenditure,
which is preferred to glucose and allows glucose to become
more available for peripheral organs.
Physical exercise enhances PPARD expression, improves
cardio-respiratory fitness and decreases circulating
lipids levels [13]. In parallel with decreased liver fat accumulation
and inflammatory markers, enhanced glucose
uptake associated with physical exercise is also observed
[11,13]. Moreover, the type and duration of exercise determines
muscle mass or hypertrophy [28,29]. In our study, we found significantly increased expression
of PPARD after a training cycle of 2 months. Our
results are consistent with other authors’ results of research
on animals models [30-32]. Mice undergoing endurance
exercise showed an accumulation of PPARβ/δ protein in
muscle [15]. Further, muscle-specific over expression of
PPARD in mice enhanced muscle metabolism (fatty acid in
flux and β-oxidation) and remodeled muscle fiber type to
increase oxidative type 2a but not type 1 fibers. Those mice
also showed decreased body fat mass and thus, had smaller
fat cells. Interestingly, PPARD transgenic mice additionally
displayed increased glucose metabolism. Together, these
results obtained by Luquet et al. [15] in 2003, implicated
PPARD in muscle development and adaptive response to
exercise training. Another model, a mouse engineered to
express a constitutively activated form of PPARβ/δ (VP16-
PPARβ/δ) in skeletal muscle, showed that a PPARD-mediated
transcriptional pathway can regulate muscle fiber
specification, enabling the generation of a strain of mice
with a “long-distance running” phenotype [16,33].
As we mentioned above, our study has provided information
about training-induced changes in the expression
level of the PPARD gene in peripheral blood. It is worth
emphasizing that our results are compatible with those
obtained for mRNA expression level analyses performed
in human skeletal muscle samples [19,34].
Regular physical activity induces desirable changes
in plasma levels of HDL and LDL, respectively, and TGs.
Physical exercise helps in maintaining lipid homeostasis,
enhances glucose uptake and expenditure and also leads
to changes in fiber type composition from glycolytic (type
II b/x) to slow/fast oxidative (types I and IIa) fibers [35].
Positive effects of exercise are also seen on blood TGs, but
little specific effect is seen on LDL and total cholesterol
(TC). Abundant evidence supports the benefits of exercise
on levels of certain blood lipids (namely HDL-C and
TG) [36]. During the 8-week long training cycle we also
observed changes in lipid profile, confirming the results
of other researchers [37,38]. However, we did not find
any statistically significant differences before and after
the training cycle.
We have also documented that 8-week long training
cycles lead to changes in the absolute FAT (kg) level
(decreased). The studies of other authors also showed the
decrease in absolute FAT (kg) under the influence of physical
activity [39,40].
Many researchers showed that PPARβ/δ has been
associated with the development of obesity. In mechanism
of obesity development, PPARβ/δ activation leads
to loss of adipose mass in different mouse models of obesity
(stimulating fatty acid oxidation) [33]. Moreover, the
same effects on fatty acid oxidation have been observed
in heart muscle (improved muscle contraction) [41]. Research
suggested that high-fat-diet-induced adiposity was
strongly inhibited by activation of PPARβ/δ in adipose
tissue. Moreover, in vitro model activation of PPARβ/δ in
adipocytes and skeletal muscle cells promotes fatty acid
oxidation and utilization. The study showed that PPARβ/δ
served as a widespread regulator of fat burning and identified
PPARβ/δ as a potential target in treatment of obesity
[33]. It is very possible that the expression of this gene
may affect the body composition analysis.
Our research may provide a starting point for further
investigating interactions between PPARD gene expression
level and the lipid profile parameters. However, our
examination has some limitations caused partly by a small
study group and biological material we had at our disposal.
Due to the fact that muscle biopsy is an invasive procedure
(especially in young people), we did not have the opportunity
to directly measure mRNA expression level in skeletal
muscles. Additionally, there is only limited knowledge
about circadian clock gene regulation by PPARD and about
its role in epigenetically modified regulation of skeletal
muscle metabolism and function. Ultimately, much work
remains to be done before any clinical PPARβ/δ-based
interventions will be possible in analysis of body composition
in athletes.
|
|
|
|

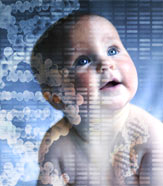

 |
Number 27 VOL. 27 (2), 2024 |
Number 27 VOL. 27 (1), 2024 |
Number 26 Number 26 VOL. 26(2), 2023 All in one |
Number 26 VOL. 26(2), 2023 |
Number 26 VOL. 26, 2023 Supplement |
Number 26 VOL. 26(1), 2023 |
Number 25 VOL. 25(2), 2022 |
Number 25 VOL. 25 (1), 2022 |
Number 24 VOL. 24(2), 2021 |
Number 24 VOL. 24(1), 2021 |
Number 23 VOL. 23(2), 2020 |
Number 22 VOL. 22(2), 2019 |
Number 22 VOL. 22(1), 2019 |
Number 22 VOL. 22, 2019 Supplement |
Number 21 VOL. 21(2), 2018 |
Number 21 VOL. 21 (1), 2018 |
Number 21 VOL. 21, 2018 Supplement |
Number 20 VOL. 20 (2), 2017 |
Number 20 VOL. 20 (1), 2017 |
Number 19 VOL. 19 (2), 2016 |
Number 19 VOL. 19 (1), 2016 |
Number 18 VOL. 18 (2), 2015 |
Number 18 VOL. 18 (1), 2015 |
Number 17 VOL. 17 (2), 2014 |
Number 17 VOL. 17 (1), 2014 |
Number 16 VOL. 16 (2), 2013 |
Number 16 VOL. 16 (1), 2013 |
Number 15 VOL. 15 (2), 2012 |
Number 15 VOL. 15, 2012 Supplement |
Number 15 Vol. 15 (1), 2012 |
Number 14 14 - Vol. 14 (2), 2011 |
Number 14 The 9th Balkan Congress of Medical Genetics |
Number 14 14 - Vol. 14 (1), 2011 |
Number 13 Vol. 13 (2), 2010 |
Number 13 Vol.13 (1), 2010 |
Number 12 Vol.12 (2), 2009 |
Number 12 Vol.12 (1), 2009 |
Number 11 Vol.11 (2),2008 |
Number 11 Vol.11 (1),2008 |
Number 10 Vol.10 (2), 2007 |
Number 10 10 (1),2007 |
Number 9 1&2, 2006 |
Number 9 3&4, 2006 |
Number 8 1&2, 2005 |
Number 8 3&4, 2004 |
Number 7 1&2, 2004 |
Number 6 3&4, 2003 |
Number 6 1&2, 2003 |
Number 5 3&4, 2002 |
Number 5 1&2, 2002 |
Number 4 Vol.3 (4), 2000 |
Number 4 Vol.2 (4), 1999 |
Number 4 Vol.1 (4), 1998 |
Number 4 3&4, 2001 |
Number 4 1&2, 2001 |
Number 3 Vol.3 (3), 2000 |
Number 3 Vol.2 (3), 1999 |
Number 3 Vol.1 (3), 1998 |
Number 2 Vol.3(2), 2000 |
Number 2 Vol.1 (2), 1998 |
Number 2 Vol.2 (2), 1999 |
Number 1 Vol.3 (1), 2000 |
Number 1 Vol.2 (1), 1999 |
Number 1 Vol.1 (1), 1998 |
|
|